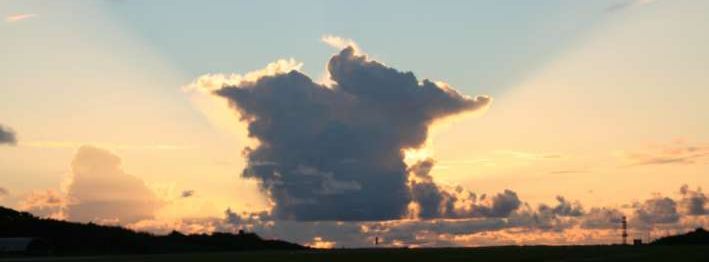
- Predictability
of intraseasonal convective perturbations in the Indo-Pacific tropical
area
- Role
of air-sea coupling in this intraseasonal variability
- Physical
origin of the intraseasonal SST variability in the western Indian Ocean
Summary
Recent
studies based on Tropical Rainfall Measuring Mission’s (TRMM)
Microwave Imager (TMI) data shows that tropical intraseasonal
perturbations of the deep convection (such as the Madden-Julian
oscillation) may be associated to Sea Surface Temperature (SST)
variations of several degrees, especially south of the equator in the
western Indian Ocean (55°E-80°E, Eq-15°S)
during boreal
winter. While this variability is partly reproduced by forced or
coupled Ocean models, the relative role of different physical processes
(warm layer formation, Ekman pumping, sub-surface cooling due to
vertical mixing, surface fluxes) in these intraseasonal SST
perturbations still has to be established.
Since
there are very few in situ observations in this region, an experimental
campaign is needed to confirm the hypotheses that can be built using
numerical modeling. The objective of the VASCO-CIRENE campaign is to
measure the impact of the different physical processes listed above on
SST perturbations from diurnal (warm layer) to intraseasonal
time-scales. This aims to better explain (i) the mechanisms of the
intraseasonal variability of the SST and (ii) the feedback of these SST
variations on the atmosphere.
The Scientific context
The
intraseasonal variability (ISV) of the deep convection has maximum
amplitude over the Indo-Pacific region and is one of the most organized
and reproducible large-scale perturbations in the Tropics. The ISV of
the convection may have a strong impact on the seasonal predictability
in the tropics. During winter, the maximum amplitude of the convective
perturbation is located between the equator and 15°S (Fig.1a).
This
perturbation propagates eastward from the West Indian Ocean to the
Central Pacific. This winter variability is generally referenced as the
Madden-Julian oscillation (MJO, see Madden and Julian 1994 for a
review). These perturbations are associated with westerly wind bursts
generating important surface flux perturbations (e.g. Weller and
Anderson 1996; Duvel et al. 2004) and that may play a role in the onset
of El Niño events (e.g. McPhaden 1999; Lengaigne et al.
2002).
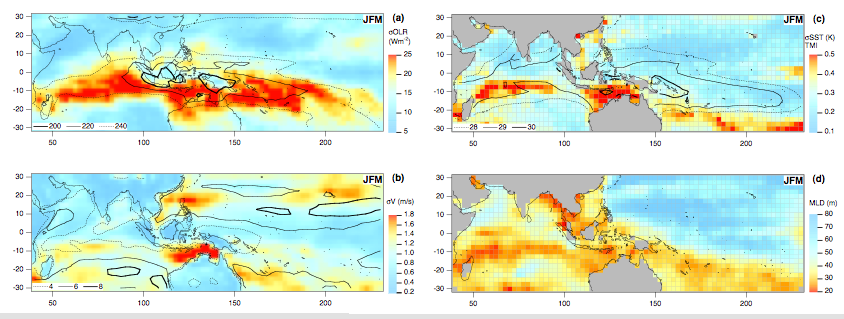
Figure
1:
JFM seasonal average (contours) and 20-90 day band standard deviation
(colors) for (a) the NOAA-OLR, (b) the NCEP surface wind module and,
(c). the TMI SST. (d). Seasonal average of the mixed layer depth from
the de Boyer Montégut (2004) climatology. (from Duvel and
Vialard, 2006)
The mechanisms
for the
generation and the evolution of the intraseasonal variability of the
deep convection over the Indo-Pacific region are not perfectly
understood. However, recent modelling studies suggest that air-sea
interactions could play an important role both during summer and winter
(e.g. Waliser et al. 1999; Inness and Slingo 2003; Maloney and Sobel
2004). Observations also have revealed SST perturbations up to 3K in
relation with the ISV of the convection in the China Sea (Kawamura
1988), in the Bay of Bengal (Sengupta and Ravichandran 2001) and in the
western Pacific (e.g. Anderson et al, 1996).
Recent satellite
measurement of the SST by the Tropical Rainfall Measuring
Mission’s (TRMM) Microwave Imager (TMI) (Wentz et al 2000)
also
revealed large SST perturbations in the Indo-Pacific region (Harrison
and Vecchi 2001; Duvel et al 2004; Duvel and Vialard 2006). These SST
perturbations are particularly strong south of the equator in the
Indian Ocean during NH winter (Fig.1c) and is associated to a
relatively thin mixed layer (Fig.1d). This determined the choice of the
region and the season for the VASCO-CIRENE experiment. These large SST
variations, identified with the TMI satellite dataset, were confirmed
by in situ data and show a potentially important role of warm layers in
the intraseasonal amplitude of the SST (Fig.2).
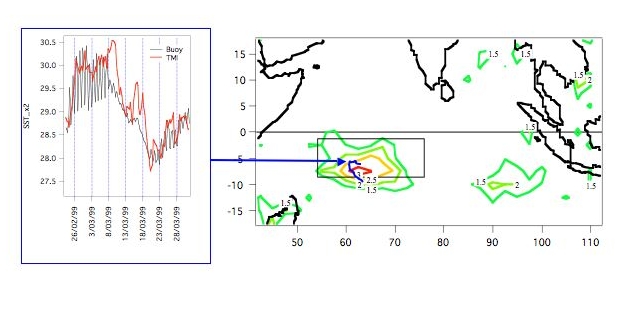
Figure
2 from Duvel et al (2004): (left) Comparison between the SST
measurement of drifting buoys and the deduced TMI SST along the path of
the buoy. TMI SST at the location of the buoy is obtained by horizontal
bi-linear interpolation and linear temporal interpolation in daily TMI
fields. The inlay shows the trajectory of the WMO 14549 between
February 25 and March 30 1999. (right) Amplitude of the TMI SST
perturbation for a strong intraseasonal event in March 1999. The path
of the buoy is indicated in blue.
The physical origin of
these large SST perturbations over the south
equatorial Indian Ocean may be due (i) to vertical and horizontal heat
transport in the ocean mixed layer, (ii) to the surface fluxes and,
(iii) to the formation of warm layers prior to the cooling event that
might contribute to enhance the SST perturbation at intraseasonal
time-scales. The shallow thermocline between 5°S and
10°S, due
to average Ekman pumping during NH winter, certainly is a fundamental
feature to explain the large observed SST perturbations. This shallow
thermocline makes cold water readily available to cool the surface by
vertical mixing or local upwelling and it also limits strongly the
depth of the mixed layer, making it more responsive to surface forcing.
This
surface
forcing
perturbation itself is due to various physical processes that may have
different phasing relative to the maximum convective activity. For the
surface heat fluxes, the western Indian Ocean during winter is more
affected by the surface wind (Fig.1b) associated to convection further
East than to the local convection (the opposite is observed over the
Eastern Indian Ocean). Duvel et al (2004) showed that the latitudinal
position of maximum SST variability was the result of a consensus
between the position of the region of maximum flux perturbation
(spanning the equator) and the region where the thermocline is shallow
(between 5°S and 12°S). Results of the forced OGCM also
showed
that the salinity perturbation induced by the strong rain under
convection and the intraseasonal Ekman pumping perturbation could play
some role in the large SST response by limiting the mixed layer
deepening induced by the wind perturbation.
Both
the scientific objectives and the geographical location of this
campaign fit into CLIVAR IOP objectives and the CIRENE region is now
recognized as a key region for the development of intraseasonal events.
Vasco-Cirene scientific
objectives